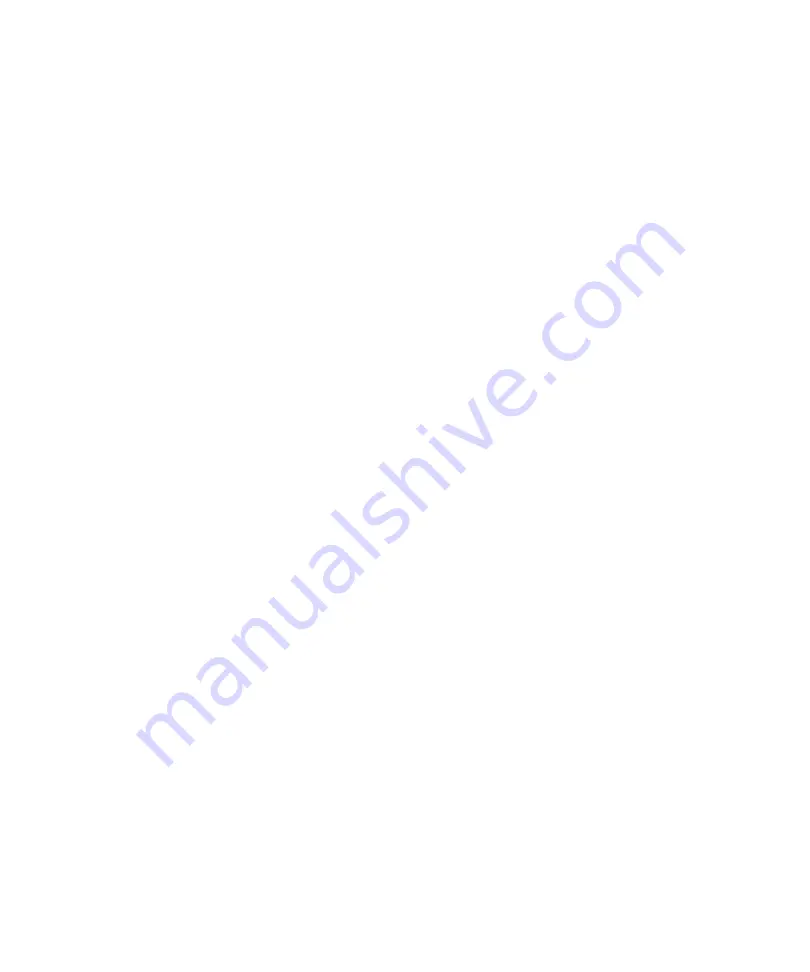
Troubleshooting
portions
of
the
cell
membrane
are
separated
from
this
tip
by
equal
access
resistance,
then
the
membrane
will
be
uniformly
voltage
clamped.
However,
many
cells
have
processes
such
as
axons,
dendrites
and
filopodia
attached
to
the
cell
body
(where
the
microelectrodes
are
usually
located).
The
membranes
of
these
processes
are
separated
from
the
cell
body
by
an
axial
access
resistance
whose
value
depends
on
the
distance
to
each
portion
of
the
membrane
and
the
cross
section
in
that
region
of
the
cell.
Thus
there
is
a
voltage
drop
across
the
access
resistance
that
becomes
substantial
for
distal
components
of
the
membrane.
Even
though
the
somatic
membrane
potential
may
be
well
controlled,
the
axonal
or
dendritic
membrane
potential
may
be
very
poorly
controlled.
In
these
cases,
the
time
course
of
synaptic
currents,
regenerative
currents
and
measurements
of
reversal
potentials
may
be
grossly
distorted.
As
a
general
rule,
the
voltage
clamp
is
considered
to
be
acceptable
if
the
length
of
the
attached
axon
or
dendrites
is
no
more
than
0.1
length
constants.
(Even
this
short
length
will
cause
significant
distortion
of
fast
currents.
See
Figure
7
in
Rall
and
Segev,
1985).
Calculation
of
the
length
constant
for
a
cell
is
complicated
since
it
depends
on
the
geometry
of
the
particular
cell
under
investigation.
Some
of
the
common
ways
to
avoid
the
problems
of
poor
space
clamping
are
as
follows:
Restrict
investigations
to
spherical
cells.
Many
cultured
cells
are
convenient.
Ligate
attached
axons.
For
example,
the
axon
of
large
molluscan
neurons
can
be
tied
off
with
nylon
thread.
Use
short
segments.
For
example,
short
segments
(100
μ
m)
of
arteriolar
syncytia
can
be
separated
from
the
arteriole
by
careful
cutting
with
a
razor
blade.
Restrict
the
range
of
the
clamp
to
a
short
segment
of
the
cell.
This
is
the
essence
of
the
“sucrose
gap”
technique
sometimes
used
on
axons.
Restrict
the
measurement
to
currents
that
are
generated
close
to
the
microelectrodes.
For
example,
the
end
plate
currents
in
muscle
fibers
165