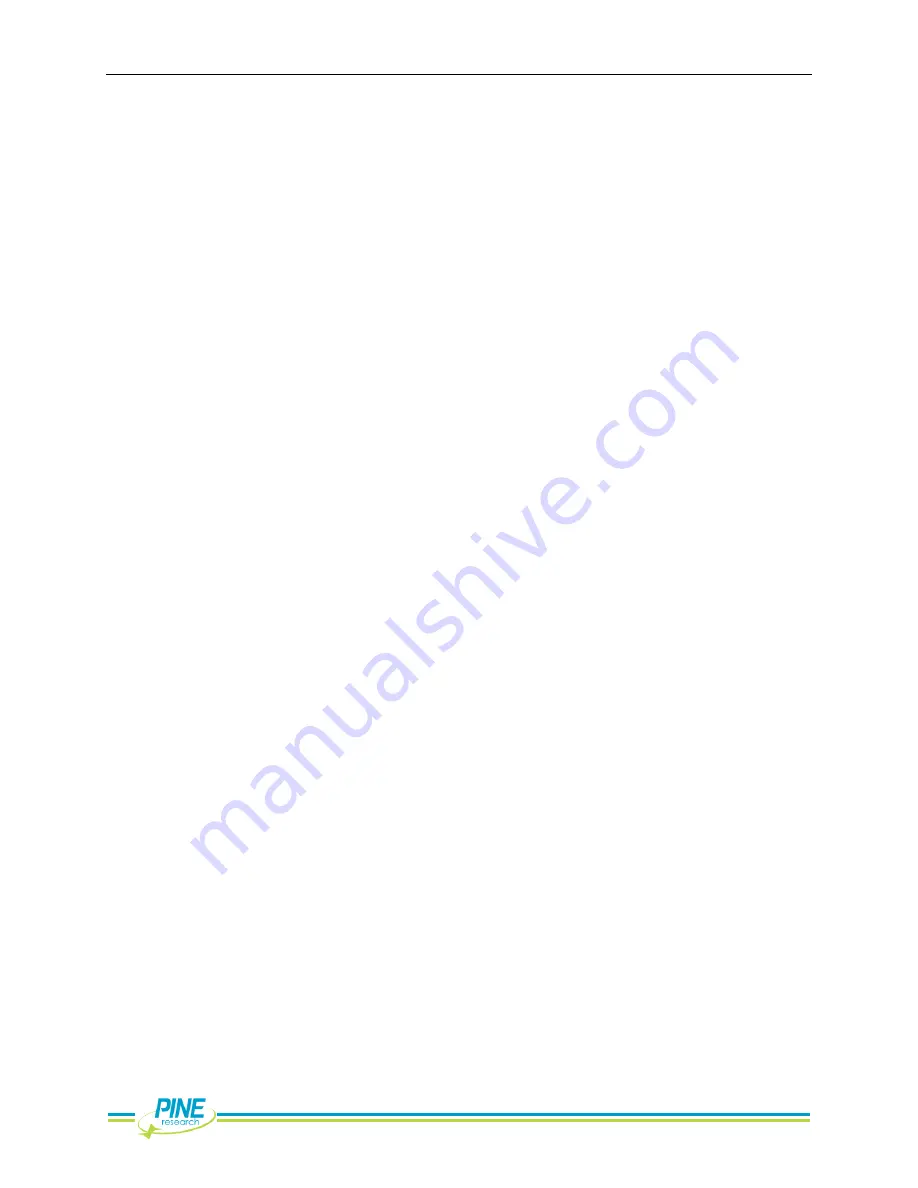
135
DRU10002 (REV 004 / JAN 2017)
in this case, especially if there is also a sloping background current. For this reason,
most experiments with rotating electrodes are conducted in solutions where only
one form of the molecule or ion is initially present.
10.4
Rotating Disk Electrode (RDE) Theory
The general theory describing mass transport at a rotating disk electrode (RDE)
was developed by Benjamin Levich at the Institute of Electrochemistry at the
Academy of Sciences of the USSR. Levich described the theory in his landmark
book,
Physiochemical Hydrodynamics
, originally published in Russian in 1952. Ten
years later, Levich’s book was
translated
[9]
from Russian to English, and the RDE
became more widely known
[11]
to Western researchers.
In the early 1960’s,
Stanley Bruckenstein
[10]
at the University of Minnesota (and his students Dennis
Johnson and Duane Napp) and Ronnie Bell
[12]
at Oxford University (and his student
John Albery) began working with rotating electrodes. Subsequent generations of
researchers expanded on this initial work, and the rotating disk electrode has
since grown into a mature tool for probing electrochemical reaction kinetics.
[13]
The laminar flow at a rotating disk electrode conveys a steady stream of material
from the bulk solution to the electrode surface. While the bulk solution far away
from the electrode remains well-stirred by the convection induced by rotation,
the portion of the solution nearer to the electrode surface tends to rotate with the
electrode. Thus, if the solution is viewed from the frame of reference of the
rotating electrode surface, then the solution appears relatively stagnant. This
relatively stagnant layer is known as the hydrodynamic boundary layer, and its
thickness (
δ
H
) can be approximated,
2
/
1
/
ω
v
3.6
δ
H
in terms of the kinematic viscosity of the solution (
v
) and the angular rotation rate
(
ω
= 2
π
f / 60
, where
f
is the rotation rate in revolutions per minute). In an aqueous
solution at a moderate rotation rate (~1000 RPM), the stagnant layer is
approximately 300 to 400
m thick.
Net movement of material to the electrode surface can be described
mathematically by applying general convection-diffusion concepts from fluid
dynamics. Mass transport of material from the bulk solution into the stagnant layer
occurs by convection (due to the stirring action of the rotating electrode). But
after the material enters the stagnant layer and moves closer to the electrode
surface, convection becomes less important and diffusion becomes more
important. Indeed, the final movement of an ion or molecule to the electrode
surface is dominated by diffusion across a very thin layer of solution immediately
adjacent to the electrode known as the diffusion layer.
Summary of Contents for MSR 636A
Page 2: ......