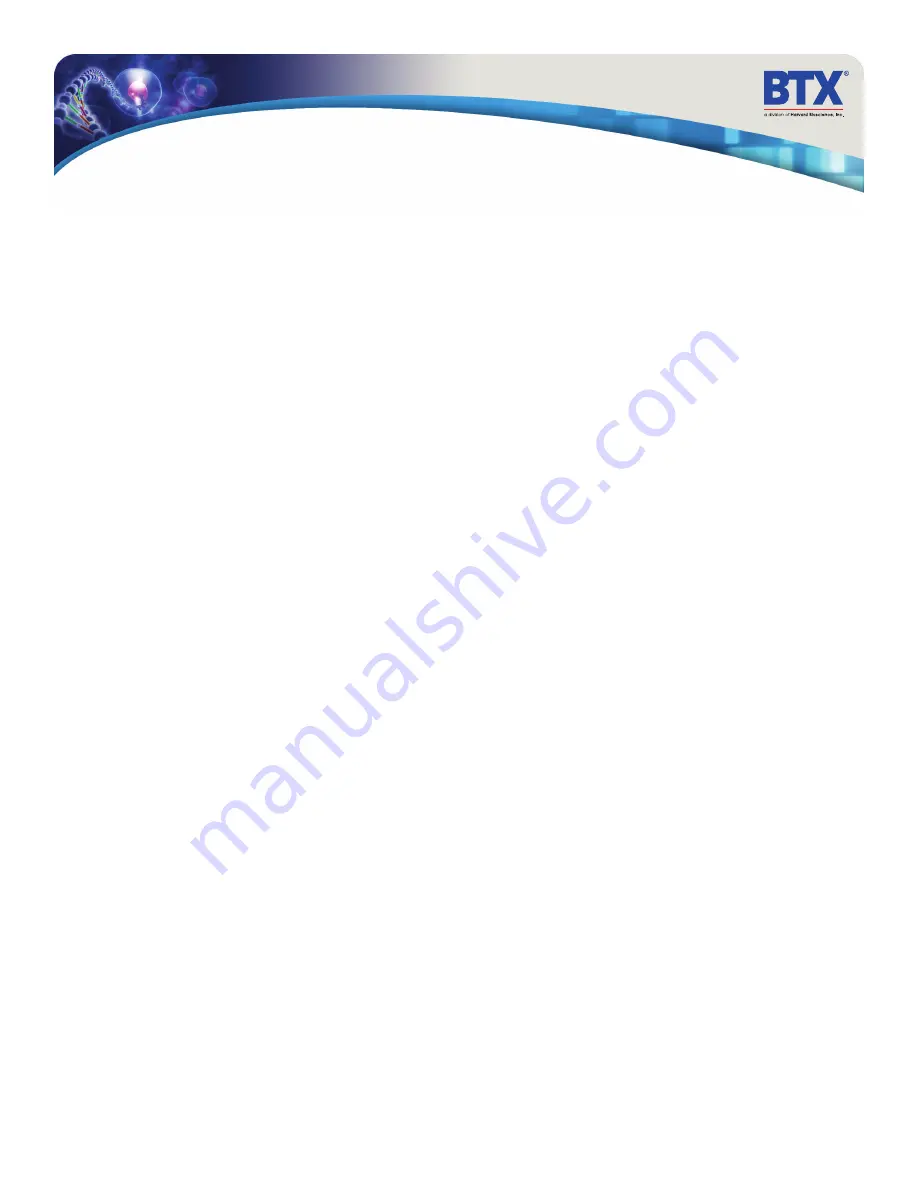
Gemini Series Electroporator User’s Manual
toll free 800.272.2775 • 508.893.8999 • www.btxonline.com
7
General Optimization Guide for Electroporation
As described, electroporation is the application of controlled
direct current (DC) electrical pulses which are applied to living
cells and tissues for a short duration of time. The pulse induces a
transmembrane potential which causes the reversible breakdown
of the cellular membrane. This action results in the permeation
or “pore formation” of the cell membrane which allows small
molecules (such as dye, oligonucleotides or peptides) and large
molecules (such as proteins, DNA and RNA) to be introduced into
the cell. During this process the cellular uptake of the molecules
continues until the pores close, which can take milliseconds to
minutes.
Optimization of the electroporation process involves several
factors. Choosing the wave form, determining field strength
and adjusting pulse length are just a few critical variables.
Other parameters which play a crucial role in optimization
include cell diameter, plasmid concentrations, temperature and
electroporation buffer.
Wave Forms
Pulse shape generally falls into two categories, square wave or
exponential decay wave:
Square Wave Pulse
Square wave pulses rise quickly to a set voltage level, maintain
this level during the duration of the set pulse length and quickly
turn off. Square waves yields higher efficiencies and viabilities in
mammalian cells. Square wave EP in
in vivo
and
ex vivo
tissues,
embryos, and plant protoplast applications yield better results in
comparison to an exponential decay wave.
Exponential Decay Wave Pulse
Exponential decay waves generate an electrical pulse by allowing
a capacitor to completely discharge. As a pulse is discharged
into a sample, the voltage rises rapidly to the peak voltage set
then declines over time. The powerful exponential decay wave
pulse is routinely used for transformation of gram-negative and
gram-positive, bacterial, yeast, plant tissues, insect cells and some
mammalian cells.
Field Strength
The field strength is measured as the voltage delivered across an
electrode gap and is expressed as kV/cm. Field strength is critical
to surpassing the electrical potential of the cell membrane to allow
the temporary reversible permeation or “pore formation” to occur
in the cell membrane. Three factors should be considered for
optimizing field strength:
1. Electrode Gap Size
2. Cell Diameter
3. Temperature
Cell Type Field Strength Ranges
Bacteria/Yeast:
3-24 kV/cm
Mammalian:
0.25-3 kV/cm
Plant:
3-12 kV/cm
Electrode Gap Size
The distance between electrodes, or “gap size” is important when
optimizing your electroporation experiment. Field strength is
calculated using voltage divided by gap size. For example, using
a 4mm gap cuvette with 500V would provide a field strength of
1.25kV/cm. If instead of a 4mm gap cuvette, a 2mm gap cuvette
was used, the voltage would have to be reduced by half or to
250V in order to maintain the same field strength of 1.25kV/
cm. It is possible to derive the voltage needed to accomplish
electroporation if the desired field strength and gap size are
known. The calculation for this is Field strength (kV) multiplied by
gap size (cm) equals voltage. For example, if a user was certain
that a 1.25 kV/cm field strength was required in a 1mm gap
cuvette the calculation would be: 1.25kV x 0.1cm= 0.125kV or
125V.
Example: A field strength of 1.25 kV/cm
4mm gap cuvette = 500V
2mm gap cuvette = 250V
1mm gap cuvette = 125V
Cell Diameter
Generally, smaller cell sizes require higher voltages while larger
cell diameters require lower voltages for successful cell membrane
permeation.
Temperature
The temperature at which cells are maintained during
electroporation effects the efficiency of the electroporation
for several reasons. The majority of mammalian cell lines are
effectively electroporated at room temperature. Samples which
are pulsed at high voltage or exposed to multiple pulses and
long pulse durations can cause the sample to heat up. These
conditions cause increased cell death and lower the transfection
efficiency. Maintaining the sample at lower temperatures can
diminish the heating effects on cell viability and efficiency. Since
electroporation causes the transient formation of pores, keeping
the cells at a lower temperature following the pulse may allow
the pores to remain open longer to allow more uptake of the
exogenous molecules. Yet lower temperatures on other cell
lines can be damaging and cause high cell mortality. This effect
is specific to each cell line and should be considered during
optimization studies. The standard pulse voltage used for cells
at room temperature will need to be approximately doubled for
electroporation at 4°C in order to effectively permeate the cell
membrane.