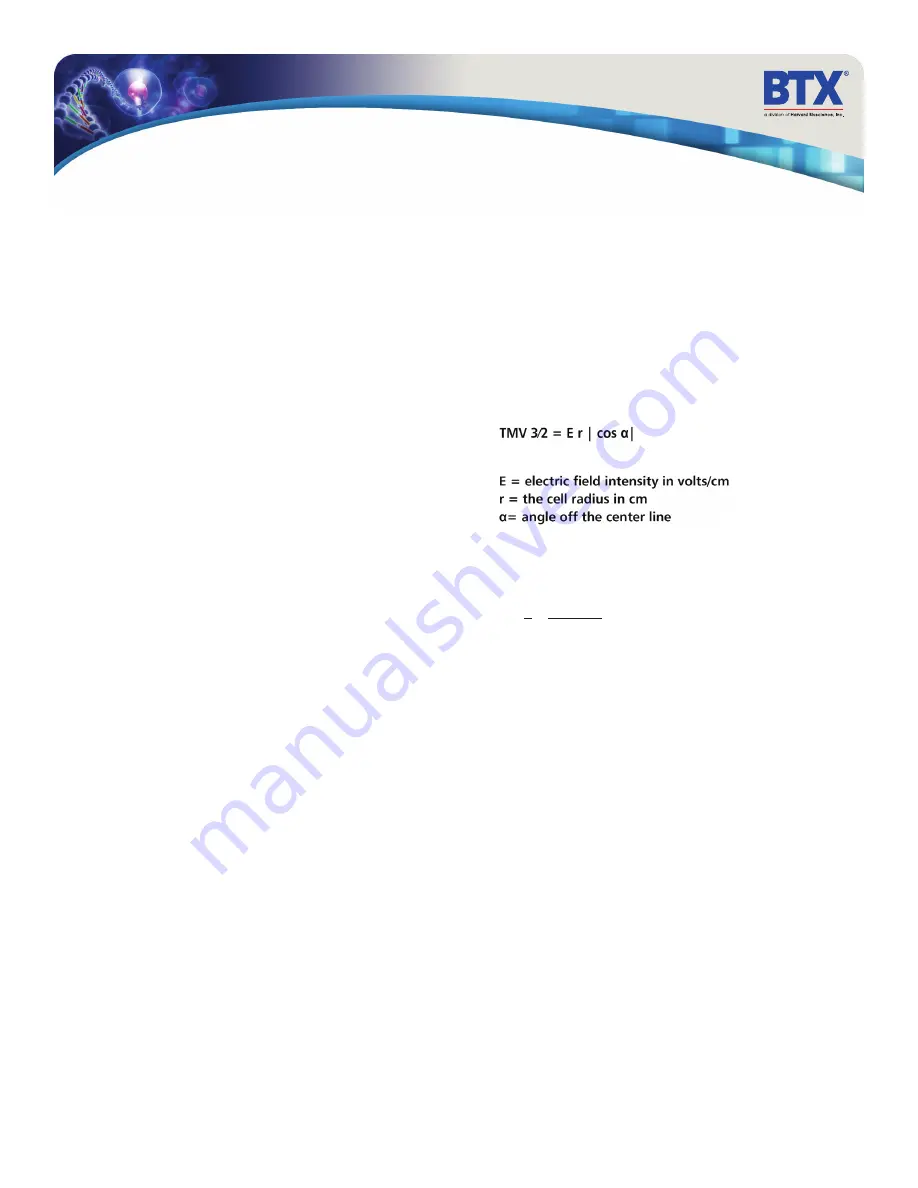
Gemini Series Electroporator User’s Manual
toll free 800.272.2775 • 508.893.8999 • www.btxonline.com
6
Electroporation Overview
DNA Delivery Into Cells Using Electroporation
General Electroporation Discussion
Electroporation is the use of a transmembrane electric field pulse
to induce microscopic pathways (pores) in a bio-membrane. Their
presence allows molecules, ions, and water to pass from one side
of the membrane to the other. When the electric field is applied the
ions inside and outside the cell membrane migrate. As the charge
builds up on either side of the membrane the membrane weakens
and the pathways form permitting material outside of the cell to
enter. If the electric field is promptly removed the pathways close
and the membrane reseals. If the electric field duration is too long
the pathways increase and the cell is killed. Efficient electroporation
depends on proper selection of electric field waveforms. The
electropores are located primarily on the membrane areas which
are closest to the electrodes. The pathways form in about a
microsecond and seal in seconds to minutes. The duration of the
electric field is tens of microseconds to tens of milliseconds.
The use of electroporation was described by Neumann in the early
1980’s. The routine use of electroporation became very popular
with researchers through the 1980’s because it was found to be a
practical way to place drugs, or other molecules into cells. In the
late 1980’s, scientists began to use electroporation for applications
in multi-cellular tissue.
In the early 1990’s Lluis Mir of the Institute Gustave-Roussy was the
first to use electroporation in a human trial to treat external tumors.
Research has shown that the induction of pathways is affected by
three major factors. First, cell-to-cell biological variability causes
some cells to be more sensitive to electroporation than other
cells. Second, for pathways to be induced, the product of the
pulse amplitude and the pulse duration has to be above a lower
limit threshold. Third, the number of pathways and effective
pathway diameter increases with the product of “amplitude” and
“duration.” Although other factors are involved, this threshold
is now understood to be largely dependent on a fourth factor,
the reciprocal of cell size. If the upper limit threshold is reached
pore diameter and total pore area are too large for the cell to
repair by any spontaneous or biological process, the result is
irreversible damage to the cell or cell lysis. Because the mechanism
of electroporation is not well understood, the development of
protocols for a particular application has usually been achieved
empirically, by adjusting pulse parameters (amplitude, duration,
number, and inter-pulse interval).
Research shows that certain experimental conditions and
parameters of electrical pulses may be capable of causing many
more molecules to move per unit time than simple diffusion. There
is also good evidence (Sukharev et al., 1992) that DNA movement
is in the opposite direction.
An additional important consideration is when the voltage pulse is
applied to the cells and medium that the amount of current that
flows is dependent on the conductivity of the material in which
the cells are located. Some material is quite conductive and severe
heating will occur if the pulse duration is too long. Therefore
long duration fields will kill cells by destroying the membrane and
heating.
The electric field in which the cells are located is produced by two
system components. The first is the voltage waveform generator
and the second is the electrode which converts the voltage into
the electric field.
As the charge accumulates at the membrane, which is a
capacitance, the voltage across the membrane increases.
voltage = capacitance charge
As charge accumulates at the membrane, the voltage across
the membrane increases. Neumann et al. (1989) described the
equation that relates the transmembrane voltage (TMV) to electric
field intensity:
where:
Pores in the membrane will begin to form as the voltage increases
from its quiescent value of a few tenths of a volt to more than 0.5
volts. To produce a TMV of 1 volt across the membrane of a cell
with 7μm radius, the required electric field intensity is:
The number of pores and effective pore diameter increase as the
product of pulse amplitude and duration increase. At the upper
limit threshold, pore diameter and total pore area become too
large for the cell to repair by any spontaneus or biological process.
The result is irreversible damage to the cell or cell lysis.
Another important point to consider is the generation of heat
during electroporation. Heat production is directly related to
current intensity which is, in turn, dependent on the conductivity
of the material through which the electric field is applied. Standard
saline solutions such as PBS and many tissue culture media are
highly conductive and, thus will generate considerable amounts of
heat when used in cell electroporation. Excessive heating can be
detrimental to cell viability. The effects of heating can be reduced
by using a low conductivity medium such as BTX’s Cytoporation
medium to resuspend cells prior to electroporation.
Although electroporation is an effective method for introducing
macromolecules onto cells, the biological mechanisms by which
cells become electroporated are not completely understood.
Therefore, the development of specific protocols for particular
applicatons is usually achieved by empirical adjustment of pulse
parameters (i.e. amplitude, duration, pulse number, and interpulse
interval).
E=
=
2
3
950 volts/cm
1
7 x 10
-4
*