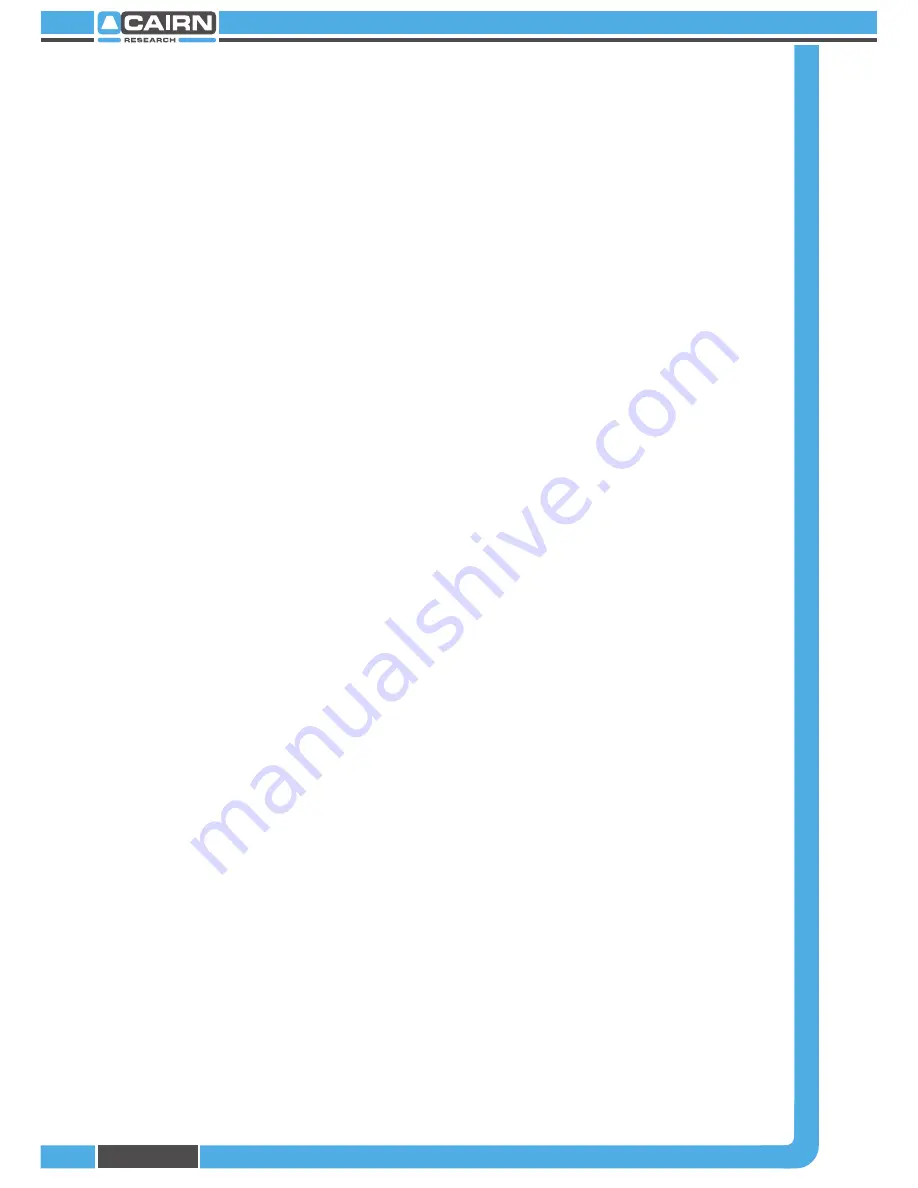
2
Installation Guide
Depending on the required configuration the LED head may have been
pre-installed in a microscope coupling (described in a separate manual), or it
may be a discrete unit. Either way we would recommend connecting the LED to
the controller box using the DIN plugs provided and pointing the LED (or output
of coupling) at a piece of white paper
(
NEVER VIEW THE LEDS DIRECTLY
)
.
Next connect the power supply to the mains, and read the following description
of the controls before verifying operation.
The front and rear panel controls are described in detail below, but if the user
bears in mind that the LED has independent controls to set whether it is on or
off and to set the intensity, and that either of these can be applied from the
front panel or externally (e.g. from a PC) then operation is quite intuitive.
‘OFF / EXT/ON’ switch
This switch allows the LED to be permanently 'ON', permanently 'OFF' or
digitally controlled 'EXT’. The digital signal is applied via the TTL (5V) 'EXT'
socket on the rear panel and allows the LED to be switched between on and off
very rapidly. When the switch is in the on or off position, this digital signal is
overridden.
‘CURRENT’ Control
This control sets the LED drive current when the 'INT/EXT' switch on the front
panel is in the 'INT'ernal position (otherwise control is via an external voltage).
The current can be set between 0 and 5A, but the protection circuit in the LED
head will usually limit maximum current.
‘LED ON’ Indicator
This indicator is illuminated whenever the corresponding LED is on. If it is lit and
there is no light at the LED head then it is probably because the intensity level
is set to zero on the front panel or the applied intensity voltage is 0V (if using
the device under external intensity control). Since its status is affected by the
digital control signals, this indicator may appear to be illuminated more or less
brightly if the applied signals are changing too rapidly to be followed by eye.
‘OVERLOAD’ Indicator
If either the steady or the transient power through the LED exceed values
preset by components within the LED head, this indicator will illuminate for
about 1 sec, during which time the drive current will be switched off to protect
the LED. Resetting is automatic, but the overload condition will keep re
triggering for as long as the power remains above these values.
For users who,
at their own risk
of course(!), wish to experiment with the
overload parameters, and/or wish to make their own custom LED heads, the
necessary information for how to do this is given in the Appendix.
‘POWER ON’ Indicator
This indicator is illuminated when the power is turned on both at the main
socket as well as the power on switch located on the rear panel.
‘INT/EXT’ Switch
This switch controls whether the current applied to the LED is set from the
front panel dial or referenced to an external voltage applied to the rear panel.
‘TTL INPUT’
This is a logic-level (i.e. 0V or +5V) input, which determines whether the LED is
on or off when in the 'EXT' mode. This input is at a logic low level by default if it
isn't connected to a suitable drive signal.
‘EXT LEVEL’
This is an external analogue input for the LED drive level where the input range
is 0 to +5V. Each change in 1V corresponds to a change in 1A.
‘ROTARY DIN’ Socket
This is an 8-pin locking DIN socket for connection to the LED head. In case you
would like to make up your own custom LED heads, the connections are
described in the Appendix.
‘POWER’ Switch
This switch needs to be turned on for the machine to operate
3
Technical summary
LED technology is continuing to develop rapidly. The first LEDs operated mainly
in the red or infra-red, and were relatively weak. However, they are now
available with wavelengths across the visible spectrum, even extending into
ultraviolet. Intensities have also increased by literally orders of magnitude, now
rivalling incandescent lamps, and in many cases competing with arc lamps.
The continual intensity improvements have caused some manufacturers to
run short of superlatives for their newer devices, which therefore have to be
called "superhyperultrabright" or some similar nonsense.
Of course, the major incentive for the development of brighter devices has
been their potential use as illumination sources, rather than just as indicators.
LED light sources have developed at a very fast rate over recent years and
Cairn have now developed their own range of LED light sources for microscopy
/ macroscopy.
The general characteristics of LEDs are as follows. Since they are a type of
diode, they have the basic characteristics of such devices, i.e. they only pass
current in one direction, and the current rises very rapidly once the voltage in
that direction exceeds a certain threshold. Ordinary silicon diodes have a
threshold voltage of about 0.65V, but LEDs have significantly higher
thresholds, e.g. around 1.2V for a standard red indicator LED. Devices
designed for shorter wavelengths tend to have higher thresholds, of maybe
around 2V or so, and in spite of the fairly steep diode characteristic they may
drop as much as 3 or 4V when driven at full power. These figures are all for a
single LED; please note that some "LEDs" may actually consist of several
devices in series,
in which case their operating voltages will be correspondingly higher. In all
cases the light output from an LED is approximately proportional to the
current, so this is the parameter that one needs to control for optical as well
as electrical reasons.
In order to drive an LED safely and reliably, a current source rather than a
voltage source is required. For low-power operation a voltage source can be
converted into a reasonable approximation of a current source by applying a
voltage significantly in excess of the device's threshold voltage in series with an
appropriate resistance. This is fine for indicator LEDs, where the current is low
and unlikely to be varied, but is far less suited for power applications. In this
case the only way to vary the power with reasonable efficiency is to vary the
series resistance, and this really isn't feasible to do directly. Instead, a
constant-current power supply with a variable current output is far more
suitable, so this is what we are using here.
However, just as a constant-voltage power supply has a maximum output
current, so does a constant-current power supply have a maximum output
voltage, and for general cost and efficiency reasons it is important to match
the current or voltage limits to match what is reasonably to be expected. The
OptoLED Lite controller therefore normally has a maximum output voltage of
about 5V. We chose this value because it can comfortably drive one LED head.
As to current ranges, indicator LEDs typically have maximum current ratings
of a few tens of mill amps at most, whereas those used for illumination may
require up to several amps. The standard drive current range for our supply is
0-5A. Although LEDs may become somewhat less efficient at higher currents,
their maximum output is ultimately limited not by optical saturation but by
thermal dissipation. Therefore, it is perfectly permissible for an LED to be
driven transiently at a higher current than the safe steady-state limit. The
extent to which this can be done, i.e. how much higher a current can be applied
for how long, will depend on the characteristics of that particular type of LED,
but information about this may be available in the device's data sheet.
Otherwise, as a rough guide, we're talking about times of up to perhaps a few
tens of milliseconds. In any case, most LEDs are relatively cheap, and they can
be destroyed without damaging the power supply, then trial and error may be
a perfectly acceptable way of exploring their safe operating limits.
Finally, a few notes about the most suitable types of LED. For general
illumination purposes, the more powerful types are clearly to be preferred.
However, for microscope illumination the situation is rather different, and in
practice better results may be achieved from using a less powerful LED here.
The reason for this is as follows. A high-magnification microscope needs to use
the condenser (or objective in the case of epi-illumination) to focus the light
onto a very small area. This requires the light going into the condenser or
objective to be nearly parallel, which is only the case if it has come from (or
behaves as if it has come from) a very compact source. Unless the output per
unit source area is also higher, any increase in source size above about 1mm
is unlikely to give brighter illumination, although it will make set-up less critical.
The chip size and configuration in the device is easily observable, and a
common configuration for the multi chip devices is to have four emitters in a
square array. For more general illumination, LED arrays with many more
devices are also available. The OptoLED Lite can drive any of these as long as
their required voltage and current requirements are within the specifications
given below.
4
Specifications
Mains input voltage 85-260V, 50-60Hz, CE compliant
Maximum output 20V
Maximum output current 5A
Response time to change in external analogue input <10usec
External analogue control voltage range 0 to+5V
Digital inputs TTL level (0V or +5V nominal)
Overload detection, parameters set by components in the LED heads
cut-out duration during overload, 1 sec nominal, auto reset
5
Appendix
The information given here is provided to allow users to construct their own
LED heads if they wish to do so. Alternatively - and perhaps more conveniently
- it will allow heads supplied by us to be customised to use other LEDs if
required.
5.1
LED Head Connections
The LED head has an 8-way locking DIN connector. The pin numbering for
these connectors can most politely be described as bizarre, having evolved
from connectors with fewer and more widely spaced pins. Therefore the pin
numbers are just given here for reference, as the following visual description is
likely to be much more helpful. Looking into the back (cable side) of the plug,
oriented with the locator slot at the bottom, you will see seven pins forming
most of a circle, with an eighth one (which actually is pin 8!) near the centre.
The connections are described in a sequence going clockwise from the pin to
the left of the locator slot, and ending with the near-central one. Heads for the
OptoLED Lite to not include a feedback photodiode so only the pins indicated in
bold are used. The heads are interchangeable between the OptoLED and the
OptoLED Lite, with the extra functionality only being active if the full OptoLED
heads are used with a full OptoLED controller.
Pin 6 Positive connection to the LED.
Pin 1 Negative (ground) connection to the LED.
Pin 4 “Array” signalling connection. If this pin is connected to a positive
voltage source, i.e. the positive supply voltage for the photodiode
amplifier on pin 7, the “array” indicator on the controller will be
illuminated, and the power readings on the meter will be reduced by
a factor of 10, to allow power levels of 20W or more to be displayed.
Otherwise this pin should be left disconnected.
Pin 2 Input connection for the overload protection network. The resistors
and capacitor comprising this network should be connected
between here and ground, i.e. pin 3.
Pin 5 Signal output from the photodiode amplifier.
Pin 3 Ground connection for the protection network and the photodiode
amplifier.
Pin 7
Positive supply (+12 to +24V, depending on the drive voltage
requirements for the LED, which are selected automatically) for the
photodiode amplifier.
Pin 8 Negative supply (-5V) for the photodiode amplifier. Note that the
controller detects the presence of an LED head by the current drawn
from here by the photodiode amplifier.
Therefore if no amplifier is fitted, a 10Kohm resistor should be connected from
here to ground (pin 3) in order to provide a dummy load.
5.2
Overload protection
The overload protection is programmed by an RC network in the plug for the
LED head. Thisnetwork forms part of a voltage divider from the measured
power signal. Normally the power signal is 100mV/W, except when an LED
array is used (detected by another connection in the LED head plug), in which
case the signal is 10mV/W to allow higher powers to be controlled.
In both cases the power signal is connected to one of two comparator inputs
(the signal input) in the OptoLED via a 100K resistor. This comparator input
also connects to the RC network in the plug via a 1K series resistor (the
resistor slightly complicates the RC network calculations but is there to
protect the comparator input), and the other end of the RC network is
connected to ground. The other comparator input (the reference input) is
connected to a 200mV internal reference input.
Overload is triggered as soon as the signal input at the comparator exceeds
the reference input; this cuts the power for about 1 second. If the protection
“network” is just a single resistor, overload will be triggered as soon as the
power exceeds the value set by this resistor. In practice, however, LEDs can be
transiently over driven, and this can be permitted by connecting a second
resistor in parallel with the first, but with a capacitor in series with the second
resistor. The peak (transient) power is set by the parallel value of the two
resistors, and this declines to the steady-state value set by the first resistor,
with a time constant that depends on the resistor and capacitor values.
The resistance value R in K ohms to set a given steady-state power level P in
watts is given by
R = 20/(0.1P-0.2) - 1
Solving for P rather than for R we obtain
P = 2 + (200/R+1)
These relations give a minimum power of 2W (or 20W for an array) when R is
infinite.
Calculated values and nearest preferred resistor values for higher powers are
as follows:
Power
Calculated
Preferred
3W
199K
200K
4W
99K
100K
5W
65.7K
68K
6W
49K
47K
7W
39K
39K
8W
32.3K
33K
9W
27.6K
27K
10W
24K
22K
12W
19K
18K
15W
14.4K
15K
17W
12.3K
12K
20W
10.1K
10K
25W
7.7K
7K5
30W
6.1K
6K2
40W
4.3K
4K3
50W
3.2K
3K3
100W
1.04K
1K
These resistor values correspond to tenfold higher power levels in array mode,
which is signalled as described in the LED head connections section of this
Appendix. Note that the highest power that can be metered is 20W (200W
for an array, although that power can't be achieved in practice), but the
overload circuitry can in theory measure power levels of up to 100W.
For many LEDs the maximum drive specification may be given as a current
rather than a power. In such cases the voltage drop across the LED at its
maximum rated current should be measured and then multiplied by the
current to determine the equivalent power level. This voltage is likely to be in
the 3-4V range for green and blue LEDs, possibly rather more for UV devices
and rather less for red ones. The permissible transient power levels may well
not be quoted, so may need to be determined by trial and error if you want to
exploit this possibility. Transient levels of 2-4 times the steady-state level, with
a decay time constant of around 10-20msec or possibly a little longer, will
probably be about as far as we can go.
For setting a higher transient power overload level, another resistor Rp, in
series with a capacitor C is connected in parallel with the resistance R
calculated as above. The steady-state overload level will be the same as before,
and the transient level will be given by the value of the two resistances in
parallel. The procedure is to choose the effective parallel resistance value, Reff,
of R and Rp to give the required transient level, and then to calculate Rp from
the relation Rp = 1/ (1/Reff - 1/R).
The time constant with which the overload power level declines from its
transient to its steadystate value is set by the capacitance C that is in series
with the parallel resistor, in conjunction with an equivalent resistance Re, to
give a time constant of ReC. The procedure for calculating C for a given time
constant T is as follows. First calculate Re, which is given by R+1K in parallel
with 100K, in series with Rp, i.e. Re = 1/{1/(R+1K) + 1/100K} + Rp. Then
calculate the required value of C from C = T/Re.
These components are connected between pins 2 (signal) and 3 (ground) of
the connector plug. The orientation is unimportant, but by convention it makes
more sense to have the capacitor on the ground side.
A practical example may be helpful in order to give some idea of the typical size
of C. Let us assume we want a transient power level of 10W, declining to a
steady-state level of 5W with a 10msec time constant. From the table we
select 68K for R to get 5W steady-state power, and then select Rp to give a
resistance of 24K (for R and Rp in parallel) to get 10W transient power. This
requires Rp to be 37K, for which the nearest preferred value of 39K will be
close enough. We now calculate Re as above to get a value of 79.8K, giving a
required value of C of about 125nF, for which either 120nF, or 100nF in
parallel with 22nF, would be close enough.
6
Technical Support
e-mail :
Web :
http://www.cairn-research..co.uk/
Address :
Cairn Research Ltd
Graveney
Road
Faversham
Kent
ME13
8UP
Telephone :
+44 (0) 1795 590 140
Fax :
+44 (0) 1795 594 510
Page 3
Summary of Contents for OptoLed Lite
Page 2: ......
Page 4: ......
Page 17: ...Page 12 ...
Page 18: ...Page 13 ...
Page 19: ...Page 14 ...
Page 20: ...Page 15 ...
Page 21: ...Page 16 ...