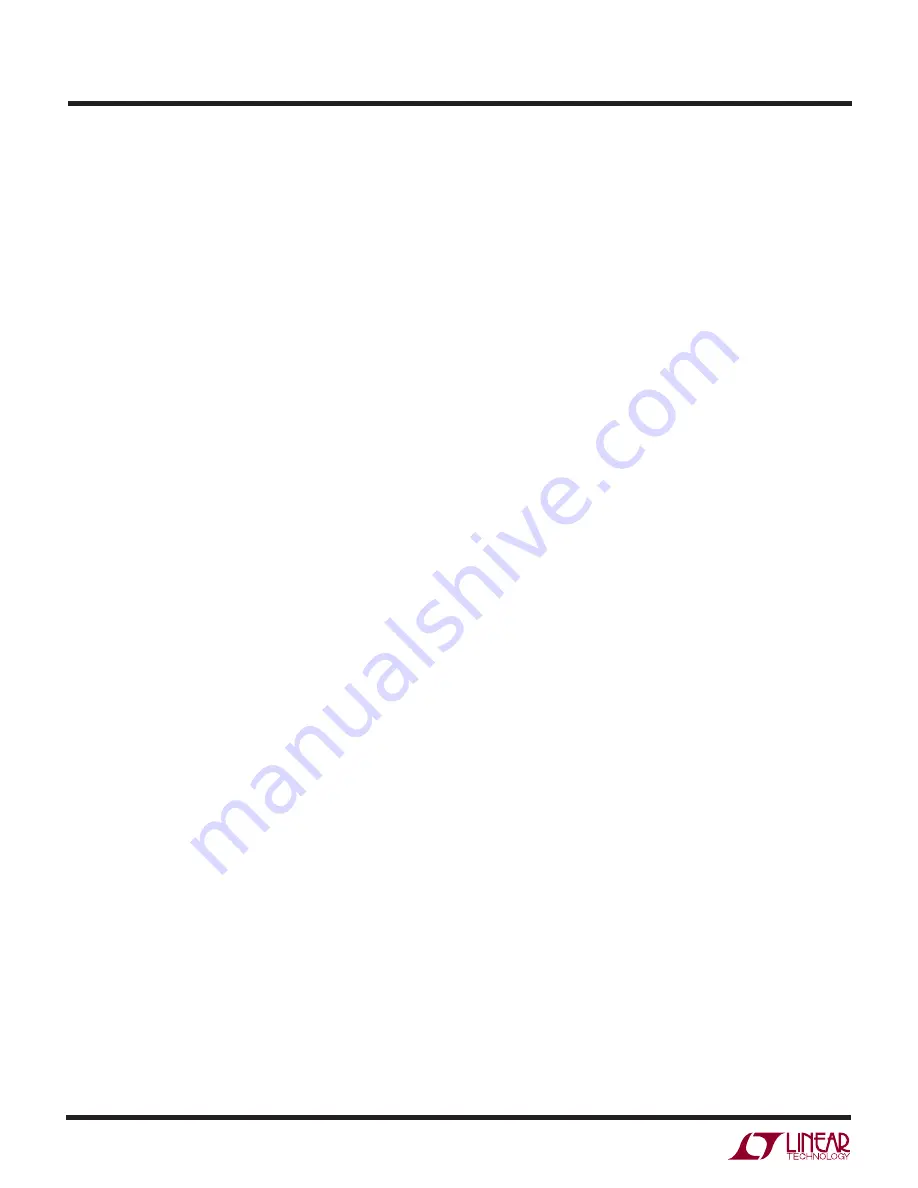
LTC3703-5
26
37035fa
MOSFET and the synchronous MOSFET. If the two
MOSFETs have approximately the same R
DS(ON)
, then the
resistance of one MOSFET can simply be summed with the
DCR resistance of L to obtain I
2
R losses. For example, if
each R
DS(ON)
= 25m
Ω
and R
L
= 25m
Ω
, then total resis-
tance is 50m
Ω
. This results in losses ranging from 1% to
5% as the output current increases from 1A to 5A for a 5V
output.
4. Transition losses apply only to the topside MOSFET in
buck mode and they become significant when operating at
higher input voltages (typically 20V or greater). Transition
losses can be estimated from the second term of the P
MAIN
equation found in the Power MOSFET Selection section.
The transition losses can become very significant at the
high end of the LTC3703-5 operating voltage range. To
improve efficiency, one may consider lowering the fre-
quency and/or using MOSFETs with lower C
RSS
at the
expense of higher R
DS(ON)
.
Other losses including C
IN
and C
OUT
ESR dissipative
losses, Schottky conduction losses during dead-time, and
inductor core losses generally account for less than 2%
total additional loss.
Transient Response
Due to the high gain error amplifier and line feedforward
compensation of the LTC3703-5, the output accuracy due
to DC variations in input voltage and output load current
will be almost negligible. For the few cycles following a
load transient, however, the output deviation may be
larger while the feedback loop is responding. Consider a
typical 48V input to 5V output application circuit,
subjected to a 1A to 5A load transient. Initially, the loop is
in regulation and the DC current in the output capacitor is
zero. Suddenly, an extra 4A (= 5A-1A) flows out of the
output capacitor while the inductor is still supplying only
1A. This sudden change will generate a (4A) • (R
ESR
)
voltage step at the output; with a typical 0.015
Ω
output
capacitor ESR, this is a 60mV step at the output.
The feedback loop will respond and will move at the band-
width allowed by the external compensation network
towards a new duty cycle. If the unity gain crossover fre-
quency is set to 50kHz, the COMP pin will get to 60% of the
way to 90% duty cycle in 3
µ
s. Now the inductor is seeing
43V across itself for a large portion of the cycle and its
current will increase from 1A at a rate set by di/dt = V/L. If
the inductor value is 10
µ
H, the peak di/dt will be 43V/10
µ
H
or 4.3A/
µ
s. Sometime in the next few micro-seconds after
the switch cycle begins, the inductor current will have
risen to the 5A level of the load current and the output
voltage will stop dropping. At this point, the inductor cur-
rent will rise somewhat above the level of the output cur-
rent to replenish the charge lost from the output capacitor
during the load transient. With a properly compensated
loop, the entire recovery time will be inside of 10
µ
s.
Most loads care only about the maximum deviation from
ideal, which occurs somewhere in the first two cycles after
the load step hits. During this time, the output capacitor
does all the work until the inductor and control loop regain
control. The initial drop (or rise if the load steps down) is
entirely controlled by the ESR of the capacitor and amounts
to most of the total voltage drop. To minimize this drop,
choose a low ESR capacitor and/or parallel multiple ca-
pacitors at the output. The capacitance value accounts for
the rest of the voltage drop until the inductor current rises.
With most output capacitors, several devices paralleled to
get the ESR down will have so much capacitance that this
drop term is negligible. Ceramic capacitors are an excep-
tion; a small ceramic capacitor can have suitably low ESR
with relatively small values of capacitance, making this
second drop term more significant.
Optimizing Loop Compensation
Loop compensation has a fundamental impact on tran-
sient recovery time, the time it takes the LTC3703-5 to
recover after the output voltage has dropped due to a load
step. Optimizing loop compensation entails maintaining
the highest possible loop bandwidth while ensuring loop
stability. The feedback component selection section de-
scribes in detail the techniques used to design an opti-
mized Type 3 feedback loop, appropriate for most
LTC3703-5 systems.
APPLICATIO S I FOR ATIO
W
U
U
U