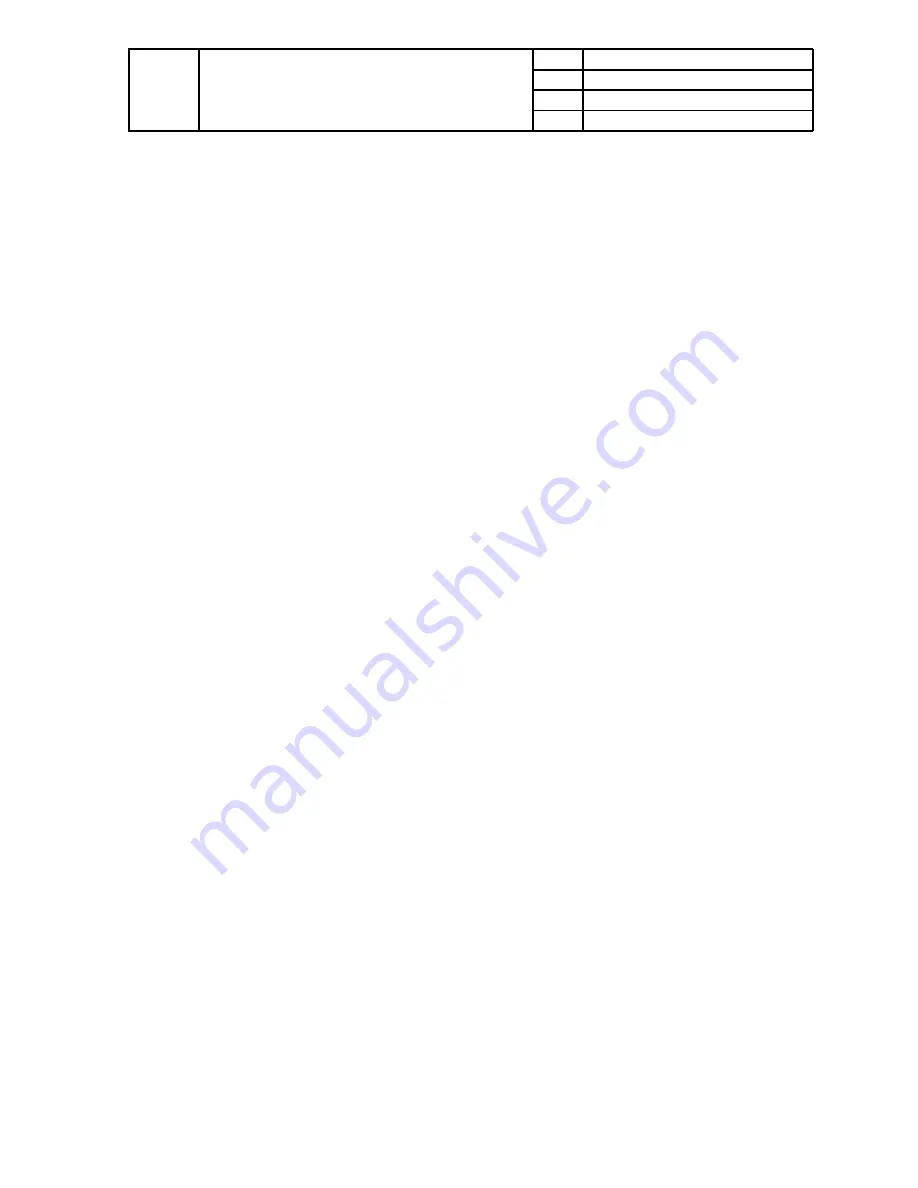
ESO
SINFONI Pipeline User Manual
Doc:
VLT-MAN-ESO-19500-3600
Issue:
Issue 1.0
Date:
Date 2005-10-19
Page:
32 of 88
6
Instrument Data Description
SINFONI data reduction often involves pair of frames: calibrations in which an “on” frame is acquired with
a calibration lamp switched on and an “off” frame recorded with the same lamp switched off, or observations
of science objects and of the sky background. Additionally, dark frames are taken as well as special fibre flat
frames used to compute optical distortions. Due to a non uniform illumination of a small number of slitlets from
the fibre link, several raw frames (usually 75) are necessary to compute the optical distortions. Each kind of raw
frame is typically associated to a single SINFONI pipeline recipe, i.e., the recipe assigned to the reduction of
that specific frame type. In the pipeline environment this recipe would be launched automatically. The recipe to
compute optical distortions takes as input several kinds of raw frames, including flats in which a few instrument
slitlets are illuminated by a fibre, and standard pairs of flat field and arc lamp frames.
In the following sections after a brief description of how most of the raw data look like, all raw SINFONI data
frames are listed, together with the keywords used for their classification and correct association. The indicated
DO category is a label assigned to any data type after it has been classified, which is then used to identify the
frames listed in the Set of Frames (see Section 4.2.2, page 22). We also provide snapshots of each of the main
raw data on frames (“off” frames snapshots are not provided as they usually look like dark frames).
6.1
Data features
In figure 6.1.0 we have represented how a possible observation target,
!
, is imaged on the detector,
!
. The 32
input source’s slices generated by the two image slicers are imaged on the detector. These are also called slitlets
and appear as vertical strips. Each slitlet has two edges, which can be seen by looking at the horizontal traces
of sky emission lines or atmospheric absorption from the hydroxyl molecule OH. Those lines also show that the
slitlets are arranged in a brick-wall pattern, to ease the detection of their edges, and that the first slitlet has a left
edge not coincident with the left border of the detector. The detector column corresponding to this edge is called
first column and it is taken as reference to measure the other 31 slitlets’ distances. This information, together
with the actual position of each slitlet edge is necessary to reconstruct the input FOV image. The detector has
a number of bad pixels and a circular defect near its center. The spatial information (both X and Y directions
on the sky) is distributed along the horizontal direction alone (the X sky coordinate in each slitlet and the Y sky
coordinate in the different slitlets) while the wavelength information is along the vertical direction, increasing
downwards. The signal from a point-like object in the FOV with a finite PSF appears as a vertical stripe in
each slitlet where its emission is relevant and it is superimposed on the sky background, more uniform along the
spatial direction except in coincidence of emission lines visible as horizontal bright stripes. During an exposure
the detector can be hit by cosmic rays.
In figure 6.1.0 we have the central plane of a coadded cube resulting from several target acquisitions observed
at different telescope positions.
In figure 6.2.0 we have several examples of raw calibration and science frames.